The Big Guide to Fusion
Nuclear fusion will not be “cracked” in a single breakthrough. Instead, there is a fixed checklist of requirements that it must work through.
This guide will walk through the checklist. I call it “The Fusion Ladder”. By the end, you will understand the important milestones in taking fusion from kid to grid.
When will we get fusion? I’ll give my opinion at the end of the piece. In the meantime, let’s look at the facts 🤓
The Fusion Ladder
Here are the steps we have to conquer to deploy fusion.
This guide will walk through each level on the fusion ladder. But first, we’ll do a 2 minute crash course in how fusion works.
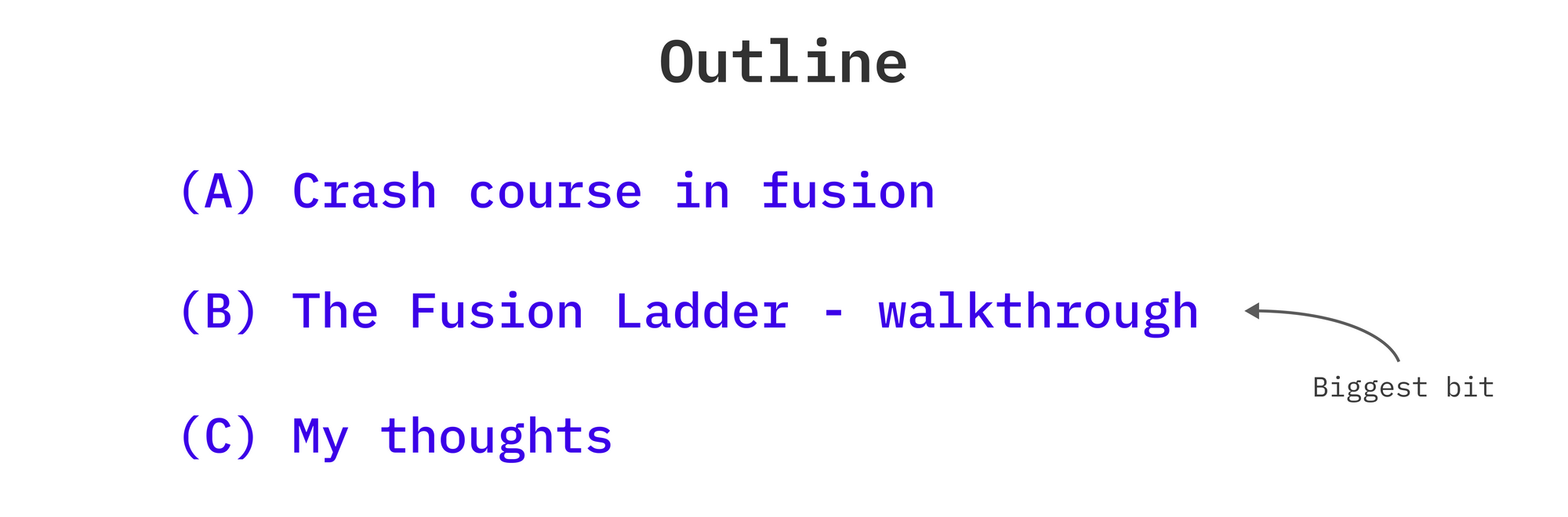
Before we start, it’s important to understand the different types of nuclear power. If you don’t know your fission from your fusion, take a read of this dropdown.
The basics - nuclear fission vs nuclear fusion
Nuclear fission and nuclear fusion are very different.
Nuclear fission means that we split big atoms apart to generate energy. All existing nuclear power plants are nuclear fission. Nuclear fission plants typically produce radioactive waste.
Nuclear fusion means that we fuse small atoms together to generate energy. No power plant using nuclear fusion has ever been built. Nuclear fusion plants may generate some waste that’s slightly radioactive, but of less concern than fission waste.
Uranium, plutonium, thorium, molten salt, and Small Modular Reactors are phrases that you might have heard chucked around. They all refer to nuclear fission.
This post is about nuclear fusion, and we won’t discuss fission.
Fusion - a 2 minute crash course
Fusion happens when we mash atoms together at high speed. This releases energy. To maximise the number of collisions between atoms, we want to:
- Make our atoms travel very fast. This usually means making them very hot (many millions of degrees).
- Confine our atoms in a small area in space.
If we can’t confine our atoms, they will just wander off. Think of a billiard ball table - without the edges, the balls wouldn’t collide much.
Doing both (1) and (2) is hard, because if you try to physically contain something that’s millions of degrees, it will melt through any material. Chocolate teapot kinda vibe.
Luckily, we’ve figured out ways to hold atoms in place without “holding” them in place. There are two mainstream approaches to confinement, and I’ll briefly explain both. The first is magnetic confinement.
Magnetic confinement
If we can’t use materials to hold our hot atoms in place, we’ll just use magnets. Sick.
There are lots of creative ideas for different shapes that we could arrange our magnets in. The most popular ones just make our particles go round in a loop ➰. The idea: if our particles keep whizzing around for long enough, eventually they'll probs bump into each other. Most of the time, they just bounce off each other, but occasionally they fuse 🤝.
The most mature magnetic loop systems are tokamaks and stellarators.
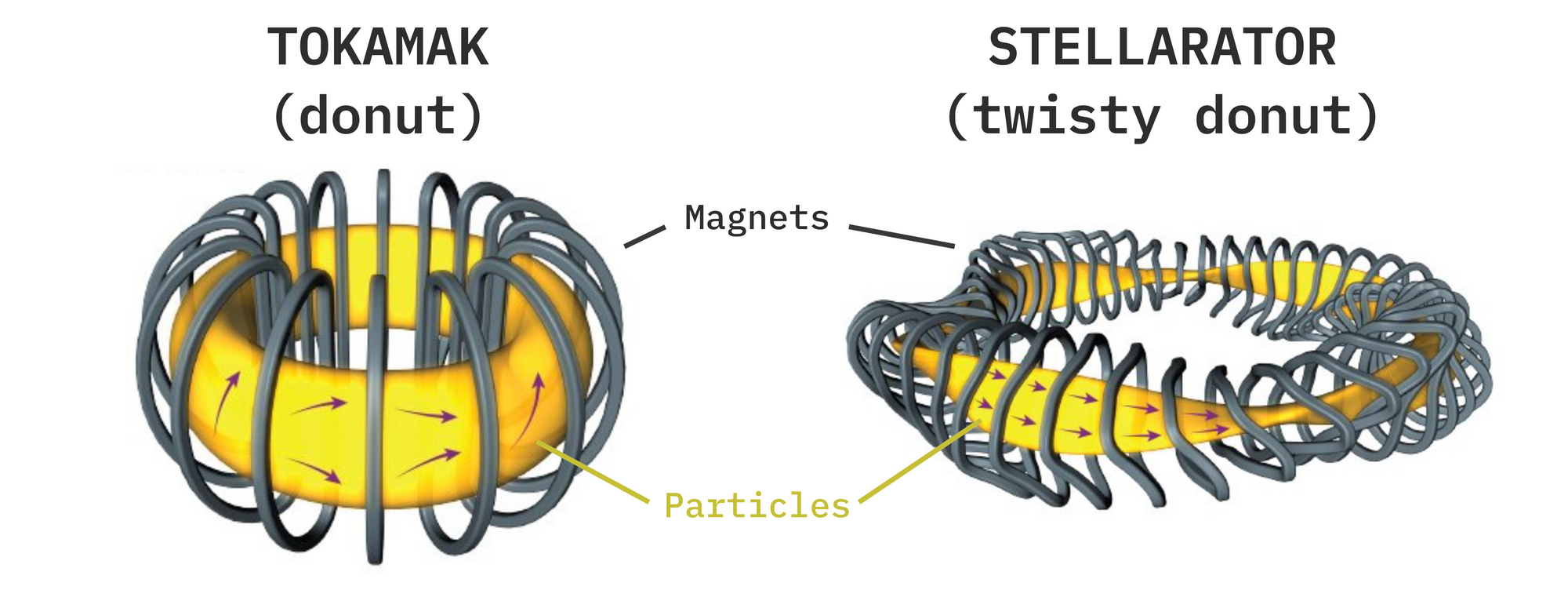
Just for fun, here’s a recording of the inside of a tokamak donut during a fusion pulse:
The pink light is created by the colder atoms that are lame and annoying. The hottest part (the best part) is in the center of the donut channel, but is invisible.
The biggest fusion experiment in the world is a tokamak. It’s called "ITER", and it’s currently being built in France. It represents the nationally funded approach to fusion, with wide international collaboration. It’s widely perceived as bureaucratic and hella slow. But boy, what an experiment it will be.
🧲 The central solenoid will be the largest superconducting magnet ever built.
💨 It has the biggest vacuum vessel in the world, weighing as much as the Eiffel tower.
🥶 It has the biggest cryoplant (cooling system) in the world, which will take two weeks to cool the reactor parts to -269°C.
🥵 The reactor’s “exhaust” will have the highest amount of heat dumped on it of any solid on earth. Each square meter will absorb up to 20MW - about the power of 60,000 UK households.
Inertial confinement
Our second big confinement method is inertial.
Inertial confinement is all about taking the atoms by surprise. We take a pellet of fuel, then heat it up and compress it very quickly. If we do this quickly enough, the centre of the pellet gets so squished that it undergoes fusion before the hot atoms have a chance to escape.
To heat up the fuel pellet, we usually use lasers, or other types of “beams and rays”.
One of the world’s most important fusion experiments is the National Ignition Facility in California. They built the biggest lasers in the world to zap a tiny pellet of fuel.
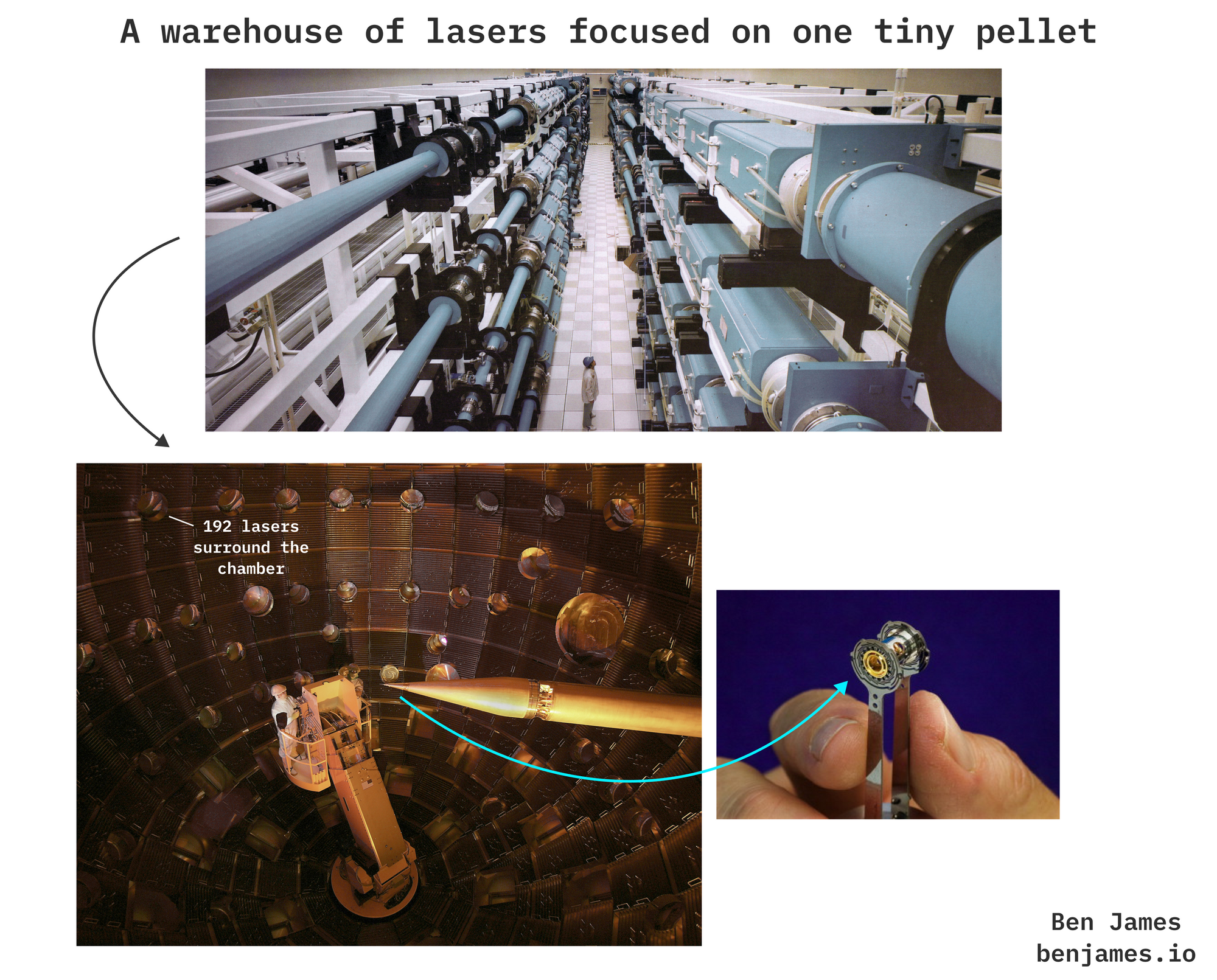
The NIF is an epic monument to science, and a gnarly physics experiment, but it’s tricky to generate electricity from infrequent mini-explosions. Also, the National Ignition Facility’s primary purpose is weapons development, not electricity generation. Bummer.
There are other methods of confinement other than magnetic and inertial, but they’re not mainstream. More on this soon.
The Fusion Ladder
Now it’s time to dive into each level on our fusion ladder. Here it is again:
Here’s the important part: every fusion method is at a different place on the ladder. The collective field-of-nuclear-fusion does not move up the ladder as one blob - instead, individual methods climb up by themselves.
LEVEL 0: Energy out > energy in (plasma)
Level 0 is about the essentials.
Remember, for our particles to fuse, first we gotta speed them up. The faster they’re moving, the more they’ll collide, and the higher the chance that they’ll fuse when they do collide. To speed 'em up, we’ll need to provide some energy. Most fusion reactors do this by heating up our particles into a hot jumbled soup called a plasma.
Obviously, if we want to make a power plant, our plasma must generate more energy than we put in to produce it. Physicists use the letter Q to measure the ratio between these quantities.
Getting to Q > 1 is hard. It has taken over 60 years of meticulous research by some of the smartest people in the world to even approach it.
Moving upwards on this graph is very tricky. As you move higher, your plasma conditions become much more extreme, and your reactor will run into unknown unknowns.
Through trial and error, the tokamak and stellarator have emerged as our most promising candidates. Not because they’re convenient or wonderful, but because they didn’t run into fundamental problems along the way. Fusion history is littered with promising designs that operated perfectly in the lower regions of this chart, but ran into tremendous difficulties as they tried to climb higher.
Most lab experiments and fusion startups cannot be plotted on the chart above, because they are too far down on the axis.
Not only this, but materials breakthroughs outside the field of fusion (best example: superconducting magnets) have provided genuine unlocks.
The legit-claims zone
Whilst all fusion methods are valid to investigate, not all methods can legitimately claim that they are likely to achieve Q > 1 in the near future.
A useful rule of thumb here is the triple product - a number that measures how favourable a plasma is. If you have to extrapolate your triple product more than 100x, there are many unknown unknowns ahead of you. You might get to Q > 1 eventually, but claiming that it’s likely to happen soon is misleading. Many fusion startups are extrapolating their success to plasma conditions more than 10,000 times more extreme.
To be clear: I’m not calling BS on the tech that new fusion startups & researchers are developing. And I’m not saying we shouldn’t work on whacky designs (we should work on far more). It’s just that there's no substitute for real experiments: you don’t get to say Q > 1 is within your reach until you’re operating very high on this chart.
Uhh, didn’t we already do level 0?
Humanity has already achieved Q > 1, in some very specific cases:
- Tests of thermonuclear bombs
- Two pulses at the National Ignition Facility (NIF) - one in 2022 and one in 2023. (The NIF is the big laser-warehouse place I showed above)
You might have read about the NIF pulses in the news. The lasers zapped a tiny pellet, which released more energy from fusion than was supplied by the lasers (Q > 1).
It was huge progress for humanity. But there’s more to this. To find out why, we need to move to level 1.
LEVEL 1: Energy out > energy in (whole plant)
Level 0 considers just the energy balance of the plasma. But it’s not the whole story.
In the case of the NIF, the pellet was zapped with 2 MJ of energy and created 3 MJ of energy. Unfortunately, the lasers required 322 MJ of electricity from the grid to create the 2 MJ that was delivered to the pellet.
It's not just the NIF. All fusion power plants will have inefficiencies where energy is lost.
So whilst getting Q > 1 for our plasma is important, it’s not enough. We need to produce a Q much bigger than 1 to make up for the inefficiencies in the rest of our system.
Here’s an example of how the energy flows through an entire tokamak power plant:
(Ps - if you’re itching to know what a “breeding blanket” is, wait for level 3)
In this tokamak example, we would need Q > 5 to compensate for the energy losses in the rest of the system.
In summary: level 1 means that we’re producing net energy at the power plant level, not just the plasma level.
LEVEL 2 - useful electricity output
We now have a reactor that’s outputting energy. But this is not the same as being a grid-ready electricity-spittin’ machine. There are two simple but crucial requirements here:
#1 - continuous energy output
To date, fusion reactors have been pulsed experiments. But for a power plant, we would like energy output that is continuous (or at least close to it). Pop the dropdown if you're feeling nerdy about this.
Some interesting things about pulsed fusion
Inertial confinement is pulsed by design. The NIF’s huge lasers discharge in under a millionth of second, then have to cool down for a few hours before going again (😎). We can easily build better lasers, but blowing things up is inherently pulsed. Of course, if we do enough pulses in quick succession, we get a near-continuous energy output (like the mini explosions in a car engine). But it’s hard to build a setup that can rapidly loop gigantic explosions under extremely sensitive conditions.
On the magnetic confinement side, there’s some interesting nuance. Powerful tokamaks have historically been pulsed experiments. ITER (the biggest tokamak currently under construction) aims to run at Q = 5 continuously, but can only run at Q > 10 for less than five minutes. The reason for this is interesting and benign:
An important part of plasma creation in a tokamak is current drive - creating a large concentric current within the plasma. This donut-current-loop confines the particles in the plasma. There are several ways to provide current drive (and we use multiple), but the simplest is a “central solenoid”. The central solenoid is just a big ol’ coil of wire that sits in the center of the donut hole. It creates a magnetic field that induces a current in the plasma.
We need a changing magnetic field to induce a constant current in the plasma. But to make a changing magnetic field, we need to supply a changing current to our central solenoid. This means we need to continuously increase solenoid current during operation. Obviously, we can’t increase the current forever, because that would be naughty. Thus, tokamaks that rely on a central solenoid for some of their current drive will always be pulsed, and not continuous. Thankfully there are other methods of current drive that are steady-state - but they’re quite complex, and come with hefty engineering.
By contrast, the stellarator does not need current drive. Its twisty magnetic fields do the hard work of confining particles, and so it does not require an induced plasma current. This means that the stellarator is an inherently steady-state design - a major selling point.
#2 - electricity, not heat
Most fusion reactors generate heat, which must then be turned into electricity. The process of doing this is simple and well understood: we’ll use a steam turbine, just like in a coal or nuclear plant. But it’s an important step because it's thermodynamically impossible to do without wasting a lot of energy. The exact efficiency depends on the operating temperature of the fusion plant, but as a ballpark, you can expect to waste 50% of your energy.
Using "alternative" fusion fuels can allow us to extract electricity directly from the plasma. But for the most promising fuel, almost all energy is generated as heat. Speaking of fuels...
LEVEL 3 - fuel supply
Ok, let’s talk about the fuel that we need for fusion. By fuel, we just mean: what specific atoms are we trying to smash together?
It turns out that there are only three or four fuel combinations that are feasible to use.
Before we discuss these, we need to learn two special words that are used to refer to different varieties of hydrogen atoms.
- Deuterium is a special type of hydrogen that has an extra neutron
- Tritium is a very special type of hydrogen that has two extra neutrons
Ok. Here are our top three fusion fuels:
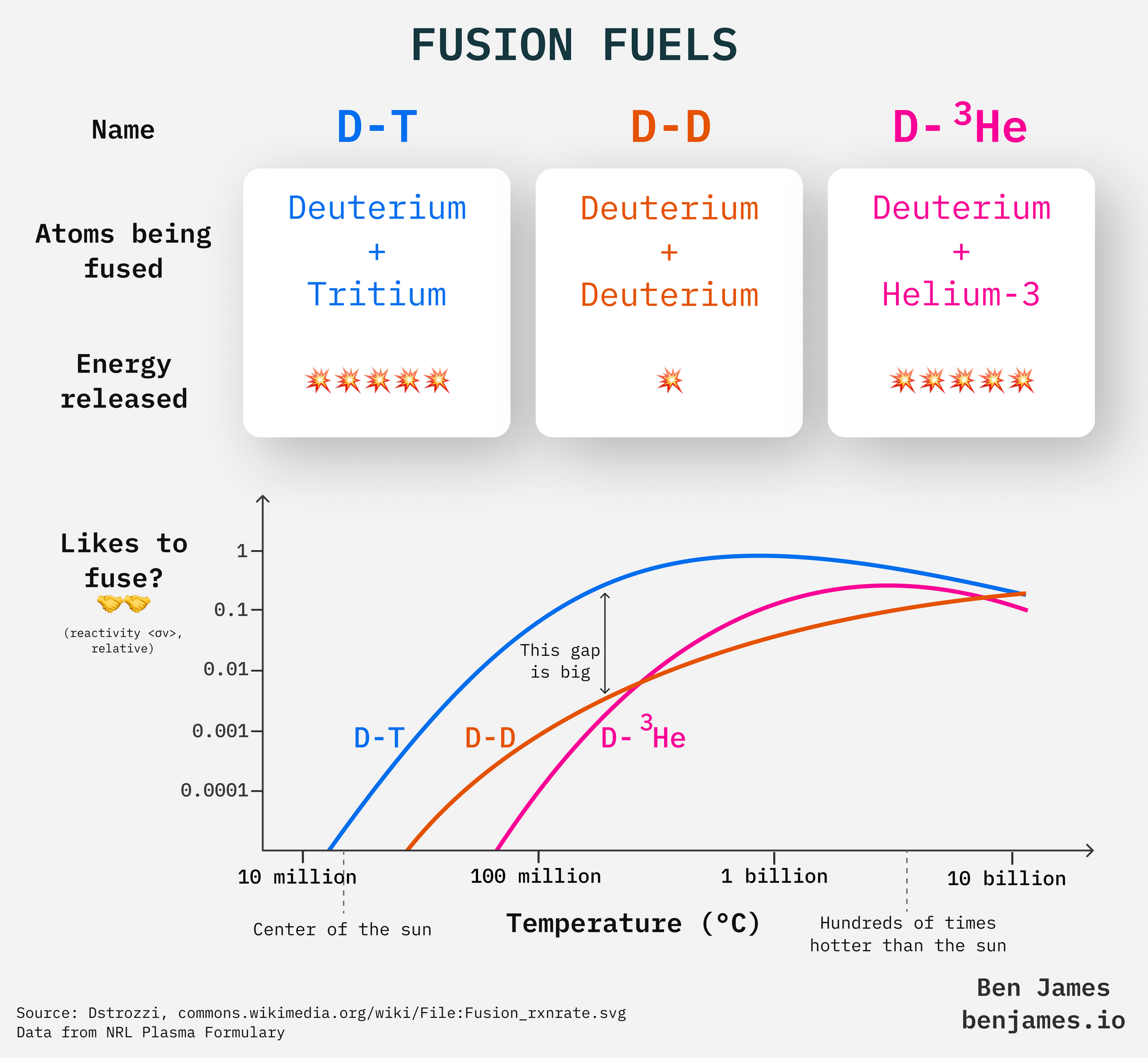
D-T slaps.
Out of the options available, fusing deuterium and tritium (D-T) is far and away the most favourable reaction. This is because:
- It has by far the biggest likelihood of fusing at “low” temperatures (still many millions of Celsius)
- It releases a lot of energy when it fuses.
D-T is the fuel that most mainstream reactors plan to use. If you plan to use another fuel, a decent chunk of people in the fusion community will consider you crazy. Looking at the graph above, to reach the same reactivity (likelihood of fusion occurring) as D-T with another fuel, your reactor might have to be 10x as hot, with even more insane engineering to boot.
Alternative fuels do have some other advantages over D-T, but it remains widely accepted that in the near term, our easiest path to high Q values will be D-T.
So where’s the nearest D-T gas station?
Sourcing Deuterium is very easy. But regrettably, dealing with Tritium is an absolute NIGHTMARE.
We currently have a stockpile of less than 40 kilograms of tritium in the entire world. Tritium is radioactive, and has a half-life of 12-years. So unless we add more of it, every 12 years our stockpiles are half as large. As we speak, tritium is busy f*cking off.
Where does tritium come from?
Unfortunately, tritium is hard to produce. Our current stockpile comes primarily from Canada’s nuclear fission reactors. These reactors happen to produce tritium as a by-product, which is a happy accident because they now sell it for $30,000 per gram 🤑. For context, Canada’s other export, Michael Bublé, is worth only $1,012 per gram.
In what many are calling a “total bummer”, Canada will close most of its tritium-producing reactors in the coming decades. Other countries may increase the tritium output from their reactors, but volumes are highly uncertain.
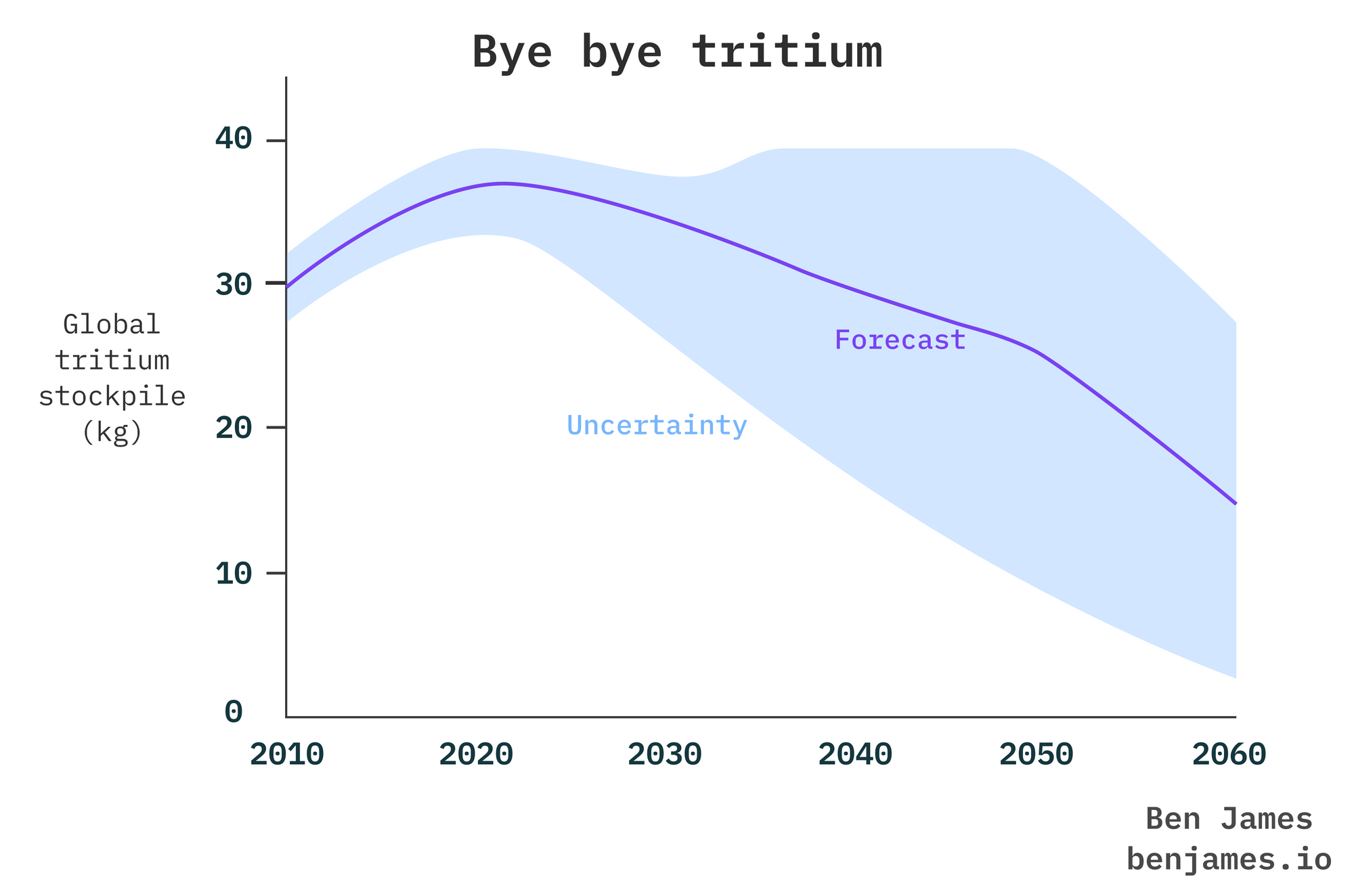
ITER will take a big chomp out of our stockpile, using 12.3 kg of tritium over its lifetime. The world’s leftover supply will be intensely fought over by research projects, fusion startups, and nuclear weapons (of which tritium is a key ingredient).
There are very serious concerns about a dwindling tritium supply for fusion research.
The plan
Luckily, we have an idea: we will build fusion reactors that not only use tritium, but also create it.
The plan is to surround a reactor with a tritium “breeding blanket”. When D-T fusion happens inside the reactor, neutrons are produced and zoom off. When they hit the breeding blanket, the neutrons interact to create tritium.
Tritium breeding blankets
Tritium breeding is a simple idea. When a neutron hits lithium-6, sometimes it creates tritium.
So, we’ll just surround our reactor with loads and loads of lithium-6, sit back and print tritium.
Lithium-6 only makes up about 7% of naturally occurring lithium, so we gotta enrich a lot of lithium first. Unfortunately, there’s basically zero supply of lithium-6 in the world. There’s an existing process for enriching lithium called COLEX, but it uses mercury and is pretty environmentally damaging. It’s effectively banned in the US due to limitations on the use of mercury in new industrial processes.
Assuming that we do manage to secure some lithium-6, we’ve still got a lot of challenges to build a breeding blanket. For every atom of tritium that enters our reactor, we want to recover or create at least one more. This means that we can’t lose any tritium in the process, which is real hard. Tritium literally soaks into the walls of a reactor. We have to do real time processing of reactor components to recover tritium. And we have to make sure that our breeding blanket surrounds as much of the reactor as possible, to capture all the neutrons. Because we’re already tight on space for instruments/beams/equipment, often at least 30% of neutrons leak.
This is a problem, and it wouldn’t be possible to close the tritium loop and get 100% recovery if it wasn’t for neutron multipliers, which give us an extra boost. Neutron multipliers take one neutron that zooms out of the reactor, and turn it into two. This means that we get more tritium out of our lithium for every neutron coming from the reactor.
Our best candidate for neutron multiplication is beryllium, which also turns out to be a pain to source. It’s toxic, and there’s not much of it. It’s estimated that one tokamak building a beryllium blanket would use about one year’s worth of our annual global supply (170 tonnes).
Breeding blanket designs are still in their early phases. ITER will not have a full breeding blanket, but will have space for “modules”, where we can experiment with different designs.
The physics of the breeding blanket is solid, but the engineering is completely untested in a real reactor. And this stuff is complex. ITER’s equipment for filtering out tritium from the breeding blanket needs its own seven storey building:
No D-T fusion startup that I’m aware of is yet building a breeding blanket. They treat it as a “we’ll figure it out later” problem. That’s understandable - but today, we have no empirical evidence that we can run a reactor producing as much tritium as it consumes.
Anecdotally, the head of a major international fusion research org recently said off the record, “guys, we forgot to think about tritium”.
Tritium means robots
There’s one last thing about tritium: when it fuses, it produces neutrons that turn the surrounding materials radioactive.
The radiation level is nowhere near as high as a conventional nuclear fission plant. But still, once you’ve put tritium in your reactor, humans can’t go in there.
This means that all reactor modifications and maintenance must be done by robots. An engineer can’t just unbolt a part or visually inspect something inside the reactor - it must be done remotely. Obviously, this makes everything very slow.
Here’s a picture of me with a maintenance robot at the JET fusion experiment in Oxfordshire, UK. I’m standing in front of the “practise reactor” - a mockup that they built purely to test the robots on.
This radioactivity also means that D-T power plants will be subject to considerable regulation.
Level 3 - conclusion
All of these problems with tritium mean that alternative “aneutronic” fuels are alluring alternatives to D-T (aneutronic fuels produce no neutrons when fused). But remember, alternative fuels have drastically lower reactivities (tendency to fuse) compared to D-T. Man, they’re hard work.
D-T vs alternative fuels is one of the greatest debates in fusion. Both sides come with astronomical challenges that justify investigating the other. I personally support the development of both.
LEVEL 4 - cost
Ok. Let’s assume that we’ve got a fusion power plant that generates electricity and has a sustainable fuel supply. This is a huge ask. But in my opinion, it’s inevitable.
Level 4 is where shit gets real. To achieve level 4, our fusion power plant must be cost-competitive with other energy sources. Level 4 is where the energy industry, state governments, manufacturing, permitting, and regulations come in. I personally believe that level 4 will be the hardest part to achieve before 2050.
Fusion will probably be expensive
As you build more of something, it usually gets cheaper. But how quickly this happens varies a lot between different energy technologies.
The power of this axis should not be underestimated. Whilst solar, wind and battery prices have plummeted during the past decade, nuclear fission costs have increased, and coal plants have stayed about the same.
Fusion reactors are complicated machines. They are also likely to be very large. This makes them subject to a lot of the same problems as nuclear fission:
- We have invented numerous designs for fission reactors that are simpler & cheaper to operate than our current reactors - on paper.
- But a new nuclear fission plant is $5bn+ on a good day. We are stuck building old designs because (1) no investor wants to build a $10bn experiment and (2) it’s a heavily regulated space.
Fusion will be less heavily regulated than fission, but comes with dramatically more complexity, and oodles more technology risk (at least for the next decades).
Can’t we have smol fusion? 👉👈
If large and complex reactors aren’t mass-producible and won’t get cheap, why can’t we make small fusion reactors instead? Why can't our cars be fusion powered?
Many fusion startups are trying to build small reactors. I applaud anyone who’s attempting it (and there are some real smart people attempting it) - but it is inordinately hard.
Small fusion reactors are tricky cos:
1) Small plasmas are hard to confine. Remember how we need to confine our plasma in a dense space so that particles can fuse?
- Big machines are great because they have a large volume (for lots of particles) and small surface area (where the particles can escape).
- Smaller reactors have a bigger surface-area-to-volume ratio, meaning that particles can escape confinement much more easily. Bad news.
2) It’s hard to span a large temperature range in a small space (magnetic confinement only)
- Superconducting magnets need cooling to -269 °C.
- Meanwhile, the inside of your plasma will be many times hotter than the sun.
- Putting very hot and very cold stuff very close is very hard. It's tricky to span the entire temperature range of the solar system in a short distance.
3) You usually need a lot of chunky auxiliary shit. For example:
- It's difficult to convert heat to electricity in a compact way. Our most efficient ways of doing this involve big turbines.
- Fusion reactors need a vacuum. Big pumps required.
- If you’re using tritium, you’ll need a ton of equipment to process and recover it from your reactor components. Remember ITER’s tritium building.
- Shielding. The deluge of neutrons emitted by any D-T reactor is harmful to humans. Big reactors are built in thick concrete buildings. A D-T reactor powering your car would need an infeasible amount of thick and heavy lead or concrete shielding.
Btw, your expensive fusion power plant is constantly destroying itself
For D-T fusion (the easiest fuel), 80% of the energy is emitted in the form of neutrons.
To be specific, hundreds of trillions of neutrons will pour out of every square centimetre of a fusion reactor every second. That’s good news, because they’re carrying the energy we need. But it’s also horrific, because they’re highly damaging.
As they pass through the reactor walls and components, the neutrons literally knock atoms out of place. This quickly damages the materials and makes them radioactive. These neutrons ain't playin'.
Plus, all the while, fast moving ions inside our plasma are escaping confinement and bonking into the side of the reactor (the pink glow in the reactor gif showed where this is happening). They’re also doing damage.
This means that all the fancy and expensive reactor components next to the plasma will need regular replacement and maintenance. This is totally Not Cool because (1) we will frequently have to buy more shit, and (2) for big fusion plants, it will take a while to do the maintenance and replacement (robots, remember?) - so we can expect significant and regular downtime.
Yo dude that's a sick kettle
Once again: for D-T fusion, 80% of the energy is emitted as neutrons. In practice, this just means heat energy.
We will then use the heat to create steam, drive a turbine, and generate electricity. So the end goal of our fusion reactor is to heat up some water.
Eli Dourado articulates the obvious relevance to cost here very well:
If you think this sounds like a complicated and expensive way to boil water, you’re right. In fact, this issue may make D-T fusion permanently uneconomical. [...]
Producing steam with coal is simple and inexpensive. It has been done for hundreds of years. We make fission unnecessarily complicated in the United States, but in principle, it, too, is simple: hold two uranium rods close together, and they make heat. We need advances in geothermal drilling to achieve steam that is hot enough anywhere on the planet to be comparable with steam from fusion, but holes in the ground are likewise simple.
In contrast, burning plasma at millions of degrees while confining it with one of the most complicated and costly machines ever built—that is an expensive way to produce steam.
Conclusions - and my opinion
Thanks for climbing up the fusion ladder with me. At the start of this guide, I promised my opinion (this is the internet, after all). My take:
There are three points that I’m confident in:
#1 The speed of fusion progress is a human decision
In the grand scheme of things, fusion funding is pretty low. If we put in more money, we could easily halve the timelines we’re working on.
The time to move up the fusion ladder should not be measured in years, but in dollars.
#2 I am optimistic because of how much we don’t know.
In researching this piece, I was surprised how many basic things we don’t yet understand about fusion. This is tremendous cause for hope.
To pick two examples:
- Modern tokamaks operate in “H-mode” - a souped-up way of getting more energy out. We discovered H-mode by accident in the 80s, and still don’t fully understand it. We might be able to enhance it even more, but we haven’t figured out the theory yet.
- The UK’s JET tokamak was built with a D-shaped cross section. It was a gamble, but turned out to work far better than expected (JET became the world’s leading fusion experiment). Since then, we’ve stuck with the D-shape because it works so well - but we should do way more experimentation with different shapes.
It’s easy to “lock on” to certain pathways that we’re confident will work. But we shouldn’t dismiss the wacky stuff, even if most of it will fail.
A good example of this is D-T fuel. Whilst it is the easiest fuel to use, and will get us to Q > 1 the fastest, its practical and economic challenges are monumental. We should investigate alternative (aneutronic) fuels heavily.
To bet against scientific progress on fusion is a bet against human ingenuity. There is simply too much that we don’t know, and our tools (in particular, computing) are improving too rapidly. However...
#3 I am pessimistic that fusion power plants will be cheap enough to get built
Physically possible does not mean commercially viable.
The science and engineering of getting to Q > 1 or Q > 10 is not where we will get stuck. Fusion’s greatest battles will not be with science, but with other energy sources and financiers.
Some investors seem to believe that once fusion has been “cracked” (Q > 1), the job is done and the technology can be rolled out. A quick peek at the early days of any electricity-generating technology reveals this to be unlikely.
Long term, fusion may win out over other energy sources, but before 2050 the competition is simply too fierce. Renewables have spent 30+ years grinding down their cost curves. Not only do they have a head start, but they will continue to fall in cost faster than fusion. It’s like trying to catch up to someone who’s running faster than you.
You could argue that fusion’s role is filling in the gaps between intermittent renewables and batteries. But the idea that we build fusion power plants to operate 10% of the time seems quite remote to me. Even under a dramatic carbon tax, fusion power plants are eye-wateringly expensive, and gas peakers are... already built.
Fusion and climate change
We need to slash emissions to zero before 2050. In my opinion, fusion is unlikely to play a role in this.
But this is no argument to deprioritise fusion development. Beyond 2050, our energy needs will still be growing. There will remain a pressing need for new, clean, power.
Fusion will quite possibly play a pivotal role in the long term development of civilisation. But to find out, we've got to survive the next decades without it.
The End
Thanks for reading - this was a big one 🙏
I had a lot of help getting up to speed on fusion, and I’m extremely grateful to everyone who gave their thoughts on this piece. Huge thanks to Theo Brown, Ana Koller, Robert Newman, Vicky McIvor, Kate Kelly, Rohan G, and Will Davison for taking time out of kicking butt to speak to me. All opinions and mistakes are mine, not theirs.
Who's Ben James?
I spend my time deconstructing climate tech, and sometimes write down my thoughts here.
I write this blog as a search query to meet interesting people. If you want to hang, please reach out 🤘.
Sometimes I run training sessions for investors who want to study climate technologies in depth. Email ben at benjames.io.
Other writing
If you enjoyed reading this, you might like some of my other posts: