Energy Fundamentals of Carbon Removal
In part one, I covered carbon capture (removing CO2 from the chimneys of power stations and industrial plants).
In this post, I cover engineered carbon removal (removing CO2 directly from the air).
Direct Air Capture (DAC) uses a lot of energy.
- Current processes use a bit more than 2000 kWh of energy to capture one tonne of CO2.
- This means that capturing one year of global CO2e emissions would require ~120,000 TWh of energy.
- 120,000 TWh is about the same amount of energy that the world uses per year. So we’d have to double the world’s energy supply just to power our giant hoover. [1] [2]
No-one is proposing that we capture all of our emissions with Direct Air Capture. But energy use is clearly critical to this technology.
In this post I’ll break down (A) where these huge energy requirements come from, and (B) what is unavoidable physics, and what is fertile ground for improvement. You won’t need a technical background to understand this post, you’ll just need to know that energy is measured in kilowatt-hours (kWh).
The basics
We need to quickly cover some basics before we dive in. I've put these in dropdowns in case you already know the drill.
Carbon capture vs carbon removal. What’s the difference?
The difference is very important.
Carbon capture refers to the removal of CO2 from point sources - the chimneys of industrial plants and power stations. This post is about carbon capture.
- When we sequester the captured CO2 underground, we call it Carbon Capture and Storage (CCS).
- When we use the CO2 (eg to make chemicals or fuels, or to extract more oil 😲), we call it Carbon Capture and Utilisation (CCU).
- CCUS (Carbon Capture, Utilisation and Storage) encompasses both and refers to the whole industry.
Carbon removal refers to the removal of CO2 from the atmosphere. Sometimes it’s called Carbon Dioxide Removal, or CDR. We can do this with nature or technology. When we do it with technology, it’s called Direct Air Capture (DAC). Post 2 in this series is about DAC.
Yo, isn’t carbon removal just a distraction from real emissions reduction?
In >90% of cases, yes it is. We need to deeply decarbonise everything that we possibly can. But we’ll still need carbon removal for (1) for the last few percent of things that are really hard to decarbonise and (2) to remove historical emissions.
The IPCC says this:
“The deployment of CDR to counterbalance hard-to-abate residual emissions is unavoidable if net zero CO2 or GHG emissions are to be achieved.”
If we are serious about the world reaching net zero emissions, then it is obvious that carbon removal will play a small role. It’s therefore important to understand what’s possible with carbon removal, so that we know where to use it or avoid it.
Is this post just about tech-bro carbon removal (DAC)? What about nature-based solutions?
Yes, this post is only about engineered carbon removal (Direct Air Capture), because this is a series on engineered methods of carbon capture. Nature-based carbon removal (trees, soils, oceans, etc) is of at least equal importance, but won’t be enough on its own.
Nature-based solutions are cheaper, and usually have environmental co-benefits. Tech-based solutions are usually more permanent, more instant, and can also provide CO2 to important processes for producing green chemicals, plastics, or fuels.
Energy visualised
Let’s compare the energy needed for carbon removal (DAC) to the energy needed for carbon capture (from point sources).
Capturing CO2 from the atmosphere uses waaay more energy than capturing it from the chimney of an industrial plant (where the CO2 is more concentrated).
Let’s first cover the fundamental physical laws at play here.
The theoretical minimum energy for carbon removal
The CO2 concentration in the atmosphere today is about 424 ppm, or 0.0424%. In a coal plant exhaust gas, the CO2 concentration will be around 11%. Therefore, separating CO2 from the atmosphere is a much harder problem, as our needle is buried in a much bigger haystack.
There is a fundamental physical limit on the minimum energy required to separate CO2 from the atmosphere, and it is 130 kWh per tonne of CO2 [3]. (Remember that for our coal plant, this only took 43 kWh per tonne).
This minimum energy cost doesn’t just apply to tech-based carbon removal. Nature-based solutions have to supply this energy too - it just comes in different forms. For example:
- In tree-planting, the energy to absorb CO2 is supplied by sunlight/photosynthesis.
- In enhanced weathering (using alkaline rocks to absorb CO2), the energy is supplied by the chemical bonds inside the rock.
After we’ve captured the CO2, we have to compress it in order to transport it or pump it underground. The theoretical minimum energy for this is 61 kWh per tonne of CO2 [4]. Adding this to the energy for separation, we end up with a total theoretical minimum energy requirement of 191 kWh per tonne of CO2.
Let’s just pause to emphasise this point: no amount of R&D, innovation or technology will allow us to remove CO2 from the atmosphere without spending at least 191 kWh per tonne of CO2.
Real life
Real life is a bummer, and we have to use a lot more energy than the theoretical minimum. In fact, we currently use more than 10x the minimum.
The leaders in deployed DAC systems are Climeworks, Carbon Engineering, and Global Thermostat. Climeworks and Carbon Engineering use processes that consume in the range of 2000 to 3000 kWh per tonne of CO2 [1]. Global Thermostat don’t publicly announce their energy numbers (it’s therefore likely that they’re not any better than the others).
Crucially, these energy requirements are not “what we got on our first try”, but long-term minimums that they expect to achieve under excellent conditions [5].
This is bad news because there are genuine reasons why DAC is harder than point-source carbon capture (more on this below). But it’s also good news, because there is a big opportunity to improve our current processes.
All energy is not equal
Things get more interesting when we consider the type of energy that these processes need.
For processes that have actually been built, like those used by Climeworks, Global Thermostat and Carbon Engineering, the majority of their energy requirement is heat, not electricity.
Most of this heat requirement comes from “regenerating” a capture material. If you read my last piece on carbon capture, you’ll be familiar with this concept. Here’s how it works for DAC.
Whilst this is greatly simplified, it is accurate to the major DAC companies today. The processes of Climeworks, Carbon Engineering, Global Thermostat and even Heirloom (“natural DAC”) are all just embellishments on this principle.
Funnily enough, it’s not absorbing the CO2 (step 1) that’s the hard part. It’s “regenerating” the capture material in step 2. The regeneration step dominates the energy consumption of current DAC technologies.
Climeworks and Global Thermostat use a solid capture material called a “sorbent” to adsorb CO2 (adsorb means that the CO2 sticks to the surface of the material), whilst Carbon Engineering uses a liquid solvent to absorb CO2. Heirloom uses crushed up calcium oxide (limestone with CO2 removed) to naturally soak up CO2.
In all of these cases, it’s temperature that’s used to regenerate the capture material (there are prototypes that use pressure, humidity, or other properties for regeneration, but they haven’t entered the mainstream yet). In fact, for both Carbon Engineering and Climeworks, 80% of their energy requirement is heat [5][6].
So if all of our mainstream DAC processes today use heat, where will we get it from? We pretty much have four options.
1. Waste heat
Industrial plants, power stations and cities all generate a lot of waste heat. Could we use it to power DAC?
Firstly, we need to consider the temperature of heat needed.
Processes that run at high temperatures will have a very hard time finding waste heat to use.
Lower-temperature processes may be able to use some, but it will be limited. If you want to nerd out here, I included some numbers in my sources doc at the bottom [9]. Suffice it to say: whilst waste heat may help get DAC off the ground, it will not power a gigaton-scale industry.
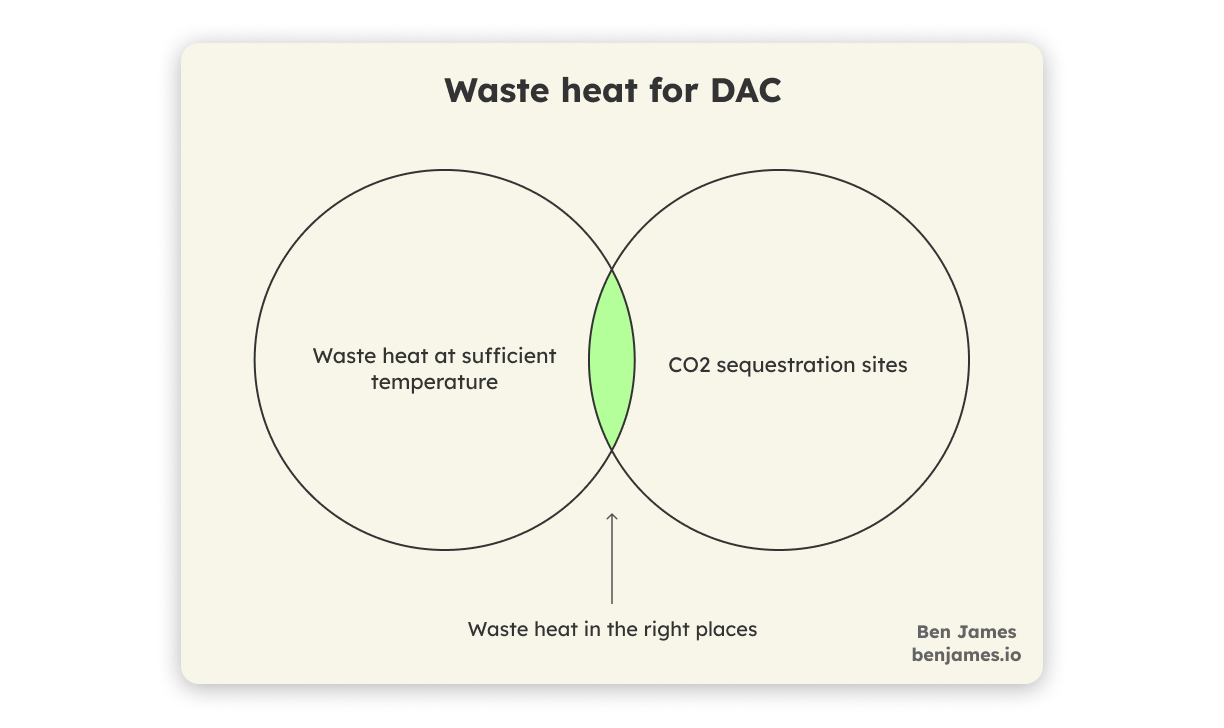
2. Fossil fuels
It seems ridiculous to mention this, but fossil fuels are unbelievably cheap sources of heat.
At first glance, the maths checks out. If you burn natural gas, you’ll get about 5000 kWh of heat for every tonne of CO2 that’s emitted [10]. So since our DAC process only needs 2000 kWh to remove a tonne of CO2, overall we’ve removed CO2. (Even more so if you capture the CO2 at the point you burn it.)
Austin Vernon puts this argument well, even if I probably don’t agree with him:
The most disruptive aspect [of DAC] would be energy usage. We'd need to ramp output up at double-digit rates because each ton of CO2 requires 2-3 MWh of energy for removal. Thankfully low-grade heat is easy to come by. There is enough energy near coal mines in Wyoming or natural gas fields in SW Pennsylvania at less than $5/MWh. [...] The key is to put the facilities at the energy sources instead of trying to move the energy. Cheap energy makes the operating costs <1% of global GDP.
If you think this sounds insane, well, people are already doing it. Carbon Engineering’s process uses natural gas for heat, and captures the CO2 from combustion. Cheap natural gas is just one reason that they're building their first large-scale plant in Texas (the other reason is Enhanced Oil Recovery).
Using fossil fuels for heat might checkout on paper, but I’m sceptical for two reasons:
(A) Methane emissions from gas extraction and coal mining are sizable, and significantly negate the benefits here. Because they vary so much geographically (and are still relatively unknown/invisible), it’s hard to definitively say whether the process is net positive or negative, but we should certainly be cautious
(B) If humanity does commit to a large-scale DAC programme, I find the image of a fossil-fuel-powered-DAC-project almost impossible to imagine a politician signing off, or getting public approval for - purely because of the optics.
3. Renewable electricity (inc. green hydrogen)
We could use renewable electricity to generate heat. There are three ways we could do this. Prices of solar, wind, and gas vary a lot around the world, so I’ve generalised the prices here.
To date, none of the currently deployed DAC plants power their heating from renewable electricity, because it’s so dang expensive. In the near-to-mid term, it’s hard to see that changing.
The critical factor here is that DAC projects are CAPEX intensive. If your plant can only run intermittently, it’s going to be difficult to make your money back.
4. Renewable heat
It’s also possible to make use of heat from the sun (concentrated solar), or geothermal heat.
Concentrated solar suffers from intermittency, whilst geothermal is expensive, but promising. Climework’s Orca project is deliberately right next to a geothermal plant in Iceland. Just a few days ago Octavia Carbon launched in Kenya, because of their abundant geothermal resources.
Why so much energy?
Ok, so we know what we’re dealing with. But still, why does DAC use so much energy in real life versus in theory? If point-source carbon capture uses only ~2.5x the theoretical minimum energy, why does Direct Air Capture use more than 10x the theoretical minimum?
Here are four reasons.
Reason #1: empirical laws of engineering
First, let’s just ignore the physics and look at the engineering. It turns out that separating stuff in small concentrations usually scales with price, in a big way. This just seems to be the empirical law of engineered systems. This graph shows a pretty solid trend.
Reason #2: increased volume of capture material
The theoretical minimum energy to separate CO2 from air may only be ~3x higher than from a coal power station. But because the CO2 concentration in the air is ~300x smaller, a lot of engineering simply has to be 300x the scale. All else being equal, you would need 300x the contact area of your capture material.
Reason #3: moving a lot of air
If you want to collect needles and your only source of them is haystacks, you’re going to have to process a lot of haystacks.
In fact, it turns out that the amount of CO2 in one metre cubed of air weighs about as much as a small sewing needle (0.75 grams). So imagine trying to collect a gigaton (1 billion tons) of needles, where for every needle that you add, you have to process one metre cubed of air. That’s a lot of air.
To illustrate the volume this entails, let’s use David Cebon’s measurement unit of a jet engine. The Rolls Royce Trent 900 jet engine is one of the largest jet engines in the world. How many of them would we need to move enough air for a large DAC system? To remove just one gigaton of CO2 per year, we would need the equivalent of 42,200 of them running full time.
Reason #4: cost optimisation
Energy is the dominant factor in the cost of DAC. But it’s not the only factor. Many parts of current DAC systems could be made more energy efficient, but only at the cost of more expensive hardware, and more complexity.
One of the leading technical authorities for a prominent DAC company told me: “A DAC system is a tradeoff between capex, opex, and reliability. We don't know where the optimum lies.”
Can we do better?
The limitations of today’s DAC systems are clear. The obvious next question is: how much can we expect DAC technology to improve in the coming decades?
My impression (as a non-expert) is that major technological breakthroughs are unlikely to come from current processes (ie heat-based processes). They will have learning curves - but will be nothing like solar/wind/batteries. I would predict that major cost reductions are likely to come from:
- Smart integration with other energy systems (eg waste heat usage)
- Entirely new ways of doing DAC.
When it comes to the latter, I’m mostly excited about one technology group in particular: electrochemistry. Electrochemical DAC is almost magical. You put two plates close together, apply electricity to them, and capture CO2. Varying the application of electricity is used for regeneration. Only electricity is required - no heat. This is hardcore science, still in development, and unproven at scale.
Electrochemistry is probably our best hope for low-energy DAC. It’s being pursued by companies like Verdox, Mission Zero Technologies, Repair-Carbon and Holy Grail (who have possibly my least favourite name for a climate startup). Some are aiming for energies less than 800 kWh / tonne of CO2.
One of the promising things about electrochemical DAC is that it is a materials challenge, to which we can apply advanced computation and machine learning. Ie, there are genuine reasons why we can build new stuff today that we couldn’t 10 years ago.
A paper on this topic wrote:
“There is vast untapped potential in the use of DFT-driven, high-throughput materials screening to accelerate the design and development of viable materials for electrochemical carbon capture and conversion.”
- Gurkan et al, 2021
It remains unclear if electrochemical DAC will make it out of the lab, but the next few years will be exciting to watch.
The end
Thanks for reading 🙏
I’m grateful to Art (who writes a killer climate tech blog) for reviewing and offering his thoughts.
Sources and footnotes are linked here.
Who's Ben James?
I spend my time deconstructing climate tech, and sometimes write down my thoughts on this blog. I’ve previously worked at World Fund, Octopus Energy, and the IPCC.
Sometimes I run training sessions for investors who want to study climate technologies in depth. Email ben at benjames.io.
Other writing
If you enjoyed reading this, you might enjoy some of my other posts: