The Big Guide to Batteries
Here are the four types of battery most relevant to the energy transition:
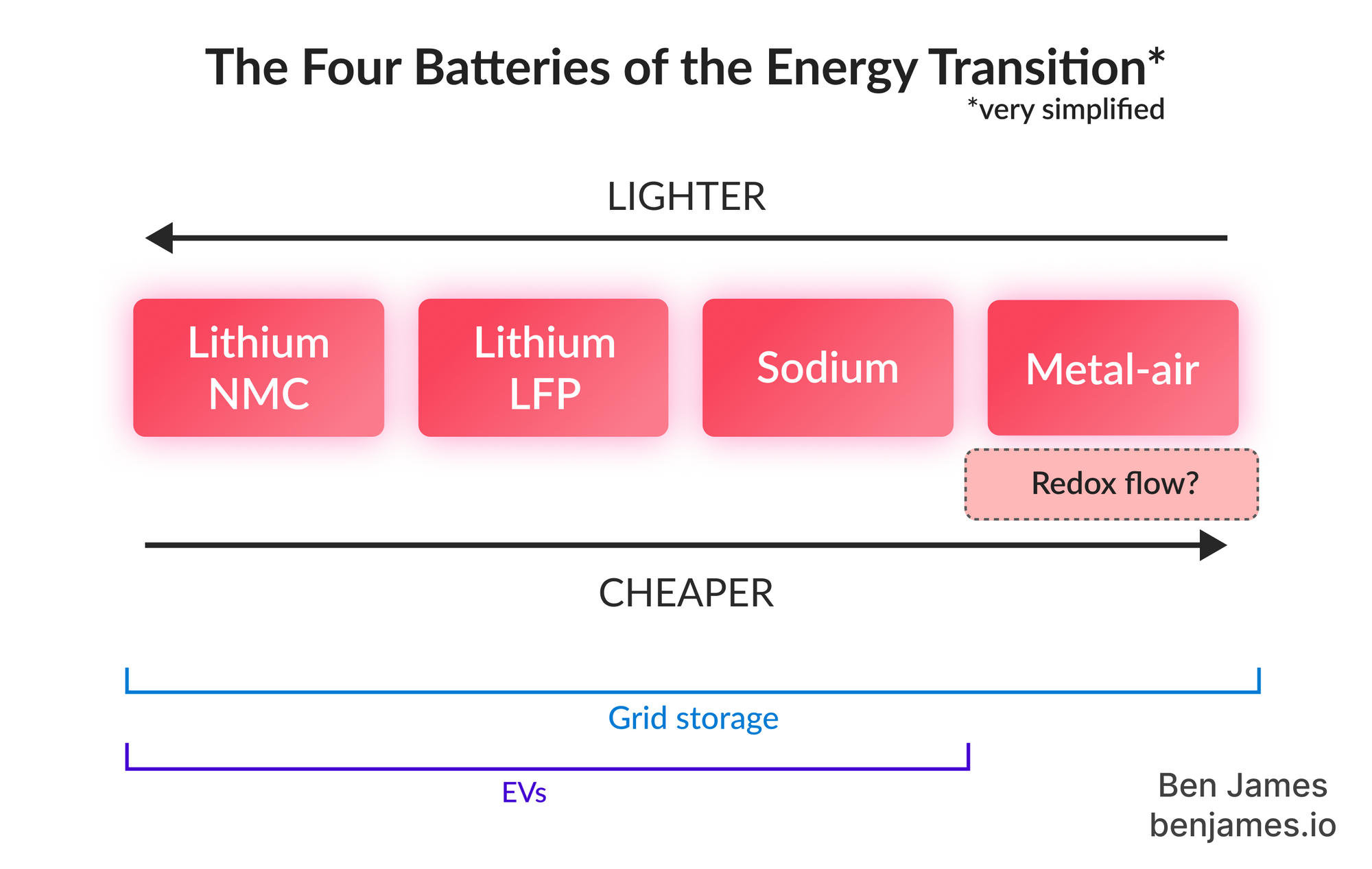
This piece is here to walk you through them all. It's intended as a concentrated onboarding guide that will orient you within the battery world of 2024 🧭. There's no chemistry, and no assumed knowledge. (By the way, this post is exclusively about electrical batteries - not thermal batteries or other types.)
In the future, our battery usage will be dominated by two things: electric vehicles and grid storage. In the first part of this guide, we’ll learn about batteries for EVs (batteries that are light and powerful). Then, we’ll move onto batteries for grid storage (batteries that can be heavier and cheaper).
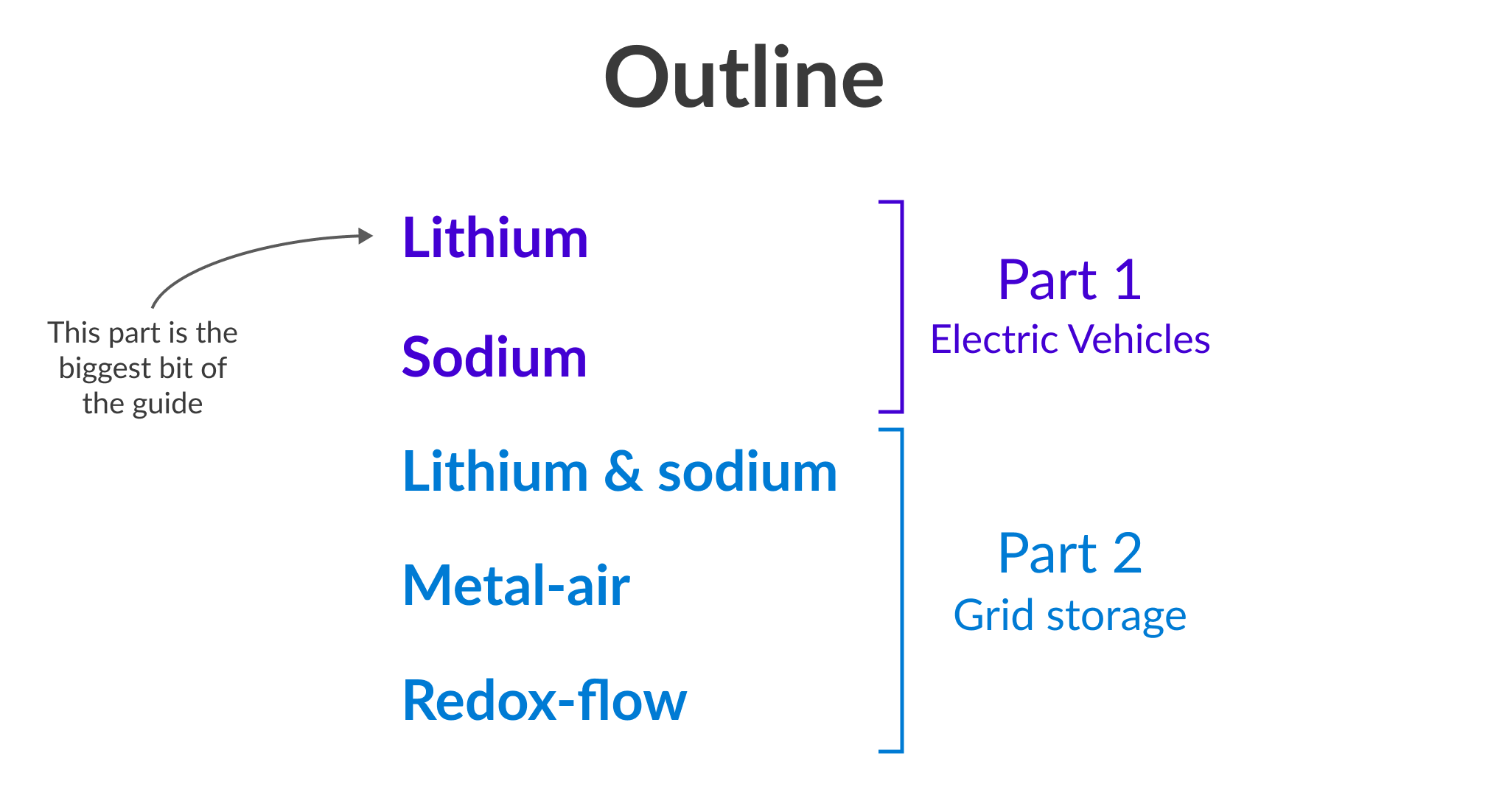
Part 1: The EV battery battle
Lithium ion
I used to think that all lithium batteries were the same. I knew that these things called “lithium ion batteries” were in our phones, laptops, EVs, and rechargeable nose hair trimmers. I figured that apart from some slight tweaks, all lithium ion batteries were pretty similar.
I was very wrong! Different types of lithium ion batteries are actually made of completely different materials. Lithium is the only big one that they have in common. In fact, there’s a fascinating battle unfolding between different types of lithium ion battery. It’s not just a story about the best battery chemistry, but one of cost, patents, and jostling superpowers.
On top of this, there’s a new kid in town. Until recently, most people thought that all EV batteries would be lithium ion. But the sodium ion battery had a breakout year in 2023. There’s a lot to learn - so let’s dive in.
I’m going to talk about this as we go through the piece in little mining boxes like this one.
Here’s a picture of how fast things are moving in EV battery land. My promise to you is that by the end of this section you’ll be able to explain all the trends happening in this graphic.
We’re going to learn about the two dominant types of lithium ion batteries: NMC and LFP. First, we need to arm ourselves with some basic terminology.
Parts of a battery
It’s useful to know about the main parts of a lithium ion battery. Basically, it’s a cheese sandwich.
Either side of the separator (cheese) are the cathode (bread 1) and the anode (bread 2). The cathode and the anode are the two large bits of the battery where the energy is stored. A lot of people are working on making bread that is lighter, smaller or cheaper, because it allows our sandwich to store more energy for less weight/space/money.
Let’s briefly go through the story of the lithium ion battery because, unbelievably, it starts with Exxon.
The birth of lithium ion in two paragraphs
In the 1970s, OPEC had put an oil embargo on the US, and people were getting nervous. Exxon decided to do some research on electric vehicle batteries, and made some progress understanding this funky thing called “intercalation”. Intercalation is a way of storing atoms in separate “host” material. If you make the atoms charged (that’s called an “ion”), you can store charge in the host material. Congratulations, you just invented the “cathode” from the picture above 🤝. Intercalation is also the namesake of the premier newsletter on batteries.
Some early lithium batteries got rapidly commercialised by a Canadian startup - but they had a tendency to catch fire. Luckily, in the early 1980s a dude named John Goodenough lived up to his name and tried using a material called lithium cobalt oxide (LCO - our first acronym 🤘) for the cathode. It worked great. Once we figured out how to make a good anode (spoiler, it’s also intercalation), Sony commercialised the first rechargeable lithium ion battery in 1991.
The crazy part is that LCO batteries are still used in smartphones and laptops today. The fundamental chemistry hasn’t changed.
A phone-powered EV?
Great, we have a stable and rechargeable lithium ion battery that’s very energy-dense. We can just make some more LCO phone batteries for EVs, right?
Phone batteries are small and cheap. EV batteries are big, and make up 30-40% of the cost of an EV.
Remember, LCO stands for Lithium Cobalt Oxide. Unfortunately, cobalt is (1) expensive, and (2) one of the most geographically concentrated and ethically troubled elements to extract.
The world’s largest cobalt reserves (45% of the world’s total) are in the Democratic Republic of the Congo, which currently produces more than 70% of the world’s supply. The appalling reality of mining and cobalt extraction in the DRC is detailed in many places online, so I will not go into it here. Suffice it to say: the less cobalt that we use, the better.
So we started replacing cobalt with a mixture of cobalt, nickel and manganese. These “diluted cobalt” cathodes are called NMC (Nickel, Manganese and Cobalt). Btw, the lithium is still in there, even if there’s not an L in the acronym. Don’t ask me why.
A star is born
It turns out that NMC lithium ion batteries are great for EVs. In fact, NMC batteries make up the majority of batteries in EVs today. The main reason for this is that they are fantastically energy dense - they carry a lot of energy for not much weight.
Up until 2015, NMC batteries had equal proportions of nickel, manganese and cobalt. This chemistry was referred to as NMC333 (meaning ~30% of each). But over time, we managed to reduce the cobalt content even more, to make chemistries like NMC532 (50% Nickel, 30% Manganese, 20% Cobalt), and nowadays, NMC811.
Whilst we’re here, you should know that NCA (Nickel Cobalt Aluminium) is a relative of NMC that uses aluminium instead of manganese. It’s a slightly lighter type of battery, but very similar to NMC. It’s been widely used by Tesla in the past, but its use is now declining.
Let’s take a look at this chart again:
We’ve got one last major type of chemistry to talk about, and that’s LFP. LFP is an old technology, and up until 2019 was dying out. However, recently it has staged a meteoric comeback that is only accelerating. This is where stuff gets really interesting.
Hi, I’m LFP and I’m a worse battery, but you’ll use me anyway 💅
LFP is lithium iron phosphate (LiFePO4, hence the acronym). LFP’s party trick is that it contains no cobalt, and no nickel - which are both expensive and geopolitically sensitive. They’re replaced by iron and phosphorus, which are abundant (and much cheaper). Iron doesn’t even make it onto the US’s chart of critical minerals.
On top of this, LFP has a much longer cycle life than NMC (the batteries degrade less over extended use). They’re also much safer!
There’s just one problem: LFP batteries are not very energy dense. To store the same amount of energy, they just weigh more. And as a battery for an EV, that’s kind of your one job.
For years, everyone had assumed that LFP’s low energy density would be a dealbreaker for EVs. In an industry obsessed with ever-increasing vehicle range, it was thought that LFP just wouldn’t be able to compete.
But in the last few years, four huge factors swung in LFP’s favour.
LFP-IS-COOL REASON 1: Safer cells = bigger cells
EV batteries are conventionally made of small cells that get assembled into modules, which get made into big complete battery packs.
Cells -> Modules -> Packs.
But because LFP is a much safer and stabler chemistry than NMC, it’s possible to produce cells that are waay bigger. If you make your cells big enough, you can skip the modules, and assemble your cells directly into a pack.
This means that you can do away with module packaging and other miscellaneous annoying shit. Your battery gets denser.
Doing away with the modules is called Cell To Pack (CTP or C2P). And although this is now happening for NMC too, LFP benefits much more because it's possible to make really huge cells.
So the punchline is this. NMC cells are way more energy dense than LFP, but by the time you’ve put them in a pack, the advantage is a lot smaller.
LFP-IS-COOL REASON 2: Patents
Previously, 95% of LFP production was in China. But in 2022, a key patent controlling LFP production outside China expired.
In early 2023, Ford announced a new $3.5bn battery plant in Michigan - to make LFP cells. Once complete it will mark the first ever large-scale US production of LFP EV batteries.
LFP-IS-COOL REASON 3: Nickel prices
Reminder: NMC is Nickel, Manganese and Cobalt. And it’s not just cobalt that’s an expensive part of NMC batteries - nickel prices have shot up too.
Whilst Russia is only the world’s third biggest producer, it has some of the highest grade (and therefore lowest cost) nickel deposits in the world.
LFP-IS-COOL REASON 4: About that range...
In the west we love to obsess about high-performance EVs with bigger and bigger ranges. But for many people who live in the city, a short range does the job just fine.
A lot of Chinese EVs have used LFP for a while, because the Chinese city commuter car market (huge) doesn’t care so much about range.
LFP-IS-COOL BONUS ROUND (slightly geeky)
There’s a ton of excitement about souping up LFP batteries by adding manganese. These batteries are called LMFP, and have the potential to significantly bump the energy density of LFP.
The idea of LMFP has been around for a while, but in 2023, big companies like CATL, BYD, Sunwoda and Eve Energy are actively working on commercialising them.
The exciting stories here:
- Chinese battery maker Gotion unveiled an LMFP cell with a density of 240 Wh / kg (compared to 160-200 Wh / kg for standard LFP).
- Tesla may begin using LMFP batteries from CATL very soon.
The future of the lithium battery
The future is bright for the lithium battery. There are numerous highly plausible routes to improve its performance even further. I'm leaving these here in optional dropdowns, as it gets a bit nerdy 🤓.
Silicon Anodes
So far we’ve mostly been talking about the cathode, because the anode hasn’t changed that much.
Since the 80s, we’ve used some form of carbon as the anode. We use graphite, and there are, broadly speaking, two ways to make it:
(1) “Natural” graphite (mined then purified)
(2) Synthetic graphite (made from fossil fuels)
Graphite is pretty good as an anode, because every six carbon atoms can “host” their own lithium ion. But silicon is an awesome anode material, because just one silicon atom can “host” three lithium ions. This means that we’d need much less silicon than graphite, and could remove a lot of the weight of the battery.
The only problem is that if you add three lithium ions to one silicon atom, well, the anode is going to get ~4x bigger. And dealing with that expansion is pretty hard.
Today we add 5-10% silicon to our graphite anodes, but we’d love to figure out how to add more. It would revolutionise mobility.
Lithium metal & solid state
Remember that thing called “intercalation” that allowed us to store lithium ions within the structure of a host material? Well, why do we need to store the lithium in a host material? Why don’t we just store it as... lithium?
This is the idea behind the lithium metal battery. Instead of having a graphite/silicon anode to store lithium within, we could just have an anode made of lithium metal. This is a lot more compact, and has the potential to significantly decrease the weight & volume of batteries, by dramatically shrinking the anode.
The challenge is that the lithium metal anode disappears and reappears as the battery is cycled. Making sure this happens evenly and non-destructively is tricky.
Lithium metal anodes have been under development for decades, and are in the process of being commercialised. In 2023, Cuberg & Northvolt announced a lithium metal cell for aviation that has been shipped to customers. The cell boasts a mass energy density of 405 Wh / kg (compared to ~300 Wh / kg for high-end NMC graphite cells), and they hope to push this to 450 Wh / kg under iteration.
One major problem with lithium metal batteries is dendrite formation. What the heck is a dendrite? Over many battery cycles, lithium can build up into spikes (a bit like stalactites), that over time can puncture the battery separator and short circuit the anode and cathode.
One way to combat this is using a solid state electrolyte. Normally, lithium ion batteries are soaked in a liquid electrolyte soup that allows lithium ions to travel between the cathode and anode. Using a solid state electrolyte is tricky, but provides the mechanical strength to prevent dendrite growth.
Solid state electrolytes have another huge benefit too: they’re much safer. So there’s a big standalone incentive to use them, even without all the lithium metal wizardry. Switching to solid state electrolytes would likely slash the (already small) number of lithium battery fires.
Unfortunately, solid state electrolytes are very tricky to manufacture. High quality and consistency are difficult to achieve, and the electrolytes are extremely fragile. This is one reason that solid state batteries (or SSBs) are also famous for being overhyped, with “solid state battery breakthrough” being one of the all-time high scorers in battery headline bingo.
Quantumscape is one of the most famous companies working on solid state lithium metal batteries. It’s been working on them since 2010, and is now a public company. In 2020 they briefly surpassed the market value of Ford, without revenue or any commercially available products 👀. It aims to begin large-scale production later this year (2023).
Wow - we talked so much about lithium ion that we're two thirds of the way through the entire piece. Now let's talk about the contenders.
Hi, I’m sodium and I’m an even worse battery, but you’ll use me anyway 💅
Ok, let’s back up for a mo. LFP is eating NMC because it’s cheaper and made from abundant materials. Even if it’s a “worse” battery (heavier for the same amount of energy stored).
What if we took this idea even further? Well, then we arrive at the sodium ion battery, sometimes called Na-ion after sodium’s chemical symbol (Na).
Why sodium? Are we just picking random elements now? It actually turns out that there are fundamental physical reasons that some elements make great batteries. Lithium is unreasonably good at being a battery material. This is because:
1. Lithium is really light (it’s actually the lightest metal)
2. Lithium really likes to donate electrons.
It just so turns out that the second best battery material is sodium, which comes in quite a bit below lithium on paper, but not too far away in practise.
And whilst you can get rid of the cobalt and nickel from lithium batteries, you’ll, err, never get rid of the lithium.
Australia currently produces the most lithium in the world. It extracts lithium from a hard rock called “spodumene”.
The Lithium Triangle is an area in the Andes which falls across Chile, Argentina and Bolivia. The huge salt flats here contain the world’s biggest resource of lithium. Lithium is not mined, but extracted from lithium-containing brines through evaporation and processing.
China also contains large lithium resources (both brine and rock), but they are lower grade. It produces a decent amount of lithium, but what is more notable is its refining capacity. More than two thirds of the world’s lithium processing happens in China, and without it, "raw" lithium is useless in batteries.
In a worst-case scenario where lithium supply dries up or is politically weaponised, there are limited options for battery replacements. For grid storage, we have a few choices (more on that below), but for EVs, the only comparable battery chemistry is sodium ion.
Sodium ion has been under development for a while, but 2023 served up a healthy dose of this-is-actually-happening announcements. Eg
- BYD announced that its Seagull EV will have a sodium-powered version, sold for $11,600. BYD recently overtook Tesla as the biggest EV manufacturer in the world.
- Swedish battery giant Northvolt recently announced a 160 Wh / kg sodium ion battery that they will soon be mass-producing
- Natron Energy recently opened the first commercial scale sodium ion factory in the US.
Sodium: the numbers
CATL’s sodium battery is expected to cost 30% less per kWh than an LFP battery. That’s huge - but the battery is too. Here’s how much bigger & heavier our sodium battery packs would be than today’s LFP systems.
Basically, we are trading off mineral abundance (and therefore, cost) with energy density (and therefore, range).
One of the most interesting things I read about sodium ion was Huiling Zhou’s post for Powerhouse. She introduces it like this:
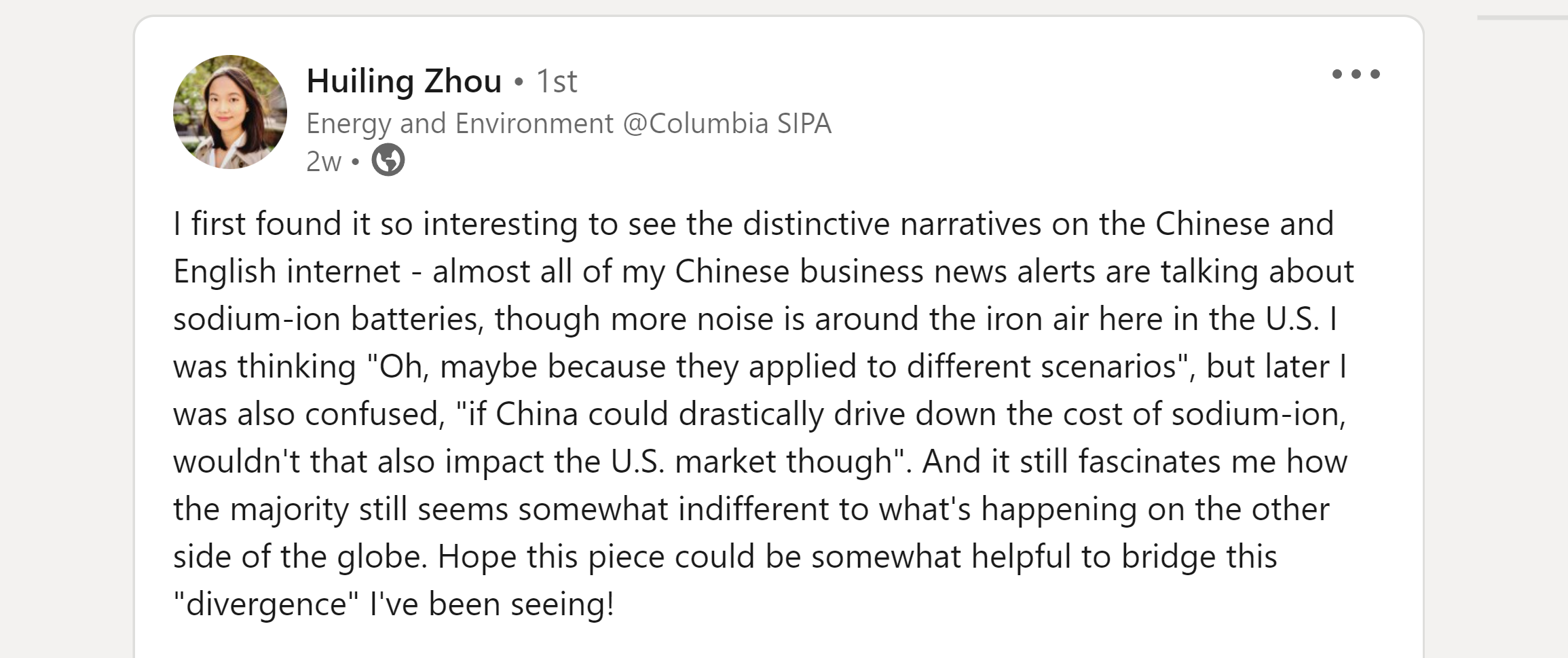
It strikes me that just a few years later, the rise of LFP may be repeating itself with sodium. The US & the west, obsessed with needlessly high-range EVs, plough ahead with high performance cells whilst China commercialises what is practical & low-cost. First LFP, and now sodium.
The last interesting thing to note about sodium is that it lives and dies by lithium prices. If lithium prices fall (from more supply coming online), then sodium’s trump card is significantly weakened. But if there’s a lithium supply crunch (like many are predicting), then sodium could really shine. In fact, it’s not difficult to use lithium kilns to process sodium. So if the lithium dries up, there might be a lot of repurposed infrastructure.
Part 2: grid storage
In part two of this guide, we’re leaving EVs behind and looking at batteries for grid storage. Don’t worry, part 2 is a lot shorter 🤠
Uhh, why can’t we just use lithium batteries for grid storage?
We can, and we do! Most grid-scale battery storage today is lithium-ion. We’re starting to see the very early stages of sodium-ion deployment here too.
But lithium batteries were originally designed for portable devices, not energy storage. They happen to be great at short-duration energy storage, because they can charge & discharge very rapidly. They’re not too expensive, and there’s also the potential to recycle old EV batteries into grid storage applications. In other words: it is very unlikely that lithium-ion will be displaced for short-duration energy storage (let’s say, up to 4 hours - but who knows where lithium’s economic cutoff will be in future).
But we also have a great need for long-duration energy storage. We need batteries that we can cycle less frequently, whilst remaining cost effective. These batteries don’t need to discharge rapidly, and they don’t need to be light. They just need to be cheap.
This is where our last two types of battery come in.
Iron-air batteries
Iron-air batteries are just one example of “metal-air” batteries - but they are of the most commercial interest.
We’ve known about iron-air batteries for ages, but no-one really bothered to build & scale them. Why? Because everyone was looking for lightweight & fast batteries.
That changed a few years ago, when Mateo Jaramillo co-founded a company called Form Energy, who set out to build a new battery specifically for multi-day energy storage. Mateo (who was previously head of stationary energy storage at Tesla) says this:
“I knew that nobody had ever tried to get to [low cost] by trading off those things...
In other words, I’m not sure anybody had ever said: this is the combination of things I’m looking for, what solves that problem?”
According to Form Energy, after a lot of experimentation, the answer to this question is iron-air batteries. When starting with the objective of low cost, the most important part is choosing abundant materials. This made iron (the cheapest metal) an obvious choice.
So here it is - I’m showing you how it works because it’s an unambiguously cool process. By rusting and “unrusting” a piece of iron, we can store electricity 🤯.
For now, the story of the iron-air battery is primarily the story of Form Energy. After years of R&D, they’ve begun construction of their first factory (it’s big), and have contracts to deliver gigawatt-hour scale projects. Incredibly, the fact that Form chose to build its factory in West Virginia may have been a key reason that the Inflation Reduction Act passed. Wild.
Form’s battery will be optimised for a duration of 100 hours, and they think that they can build it for less than $20 / kWh (lithium ion packs are yet to fall below $100 / kWh). This cost level seems to be feasible.
I’m excited to follow their progress 👀
Redox flow batteries
Out of all the batteries listed here, flow batteries have perhaps the most uncertain future. But they come up a lot, and they’re interesting because they take the concept of a battery in an entirely different direction.
A conventional battery is kind of an “all in one”. Energy is converted and stored within the same package. But a flow battery splits up the key parts. It has (1) a “storage” part of the battery, and (2) a “reactor” part of the battery.
Energy is stored in the tanks, in chemical form. When the battery needs to be charged or discharged, the chemicals are pumped to the reactor, where they interact to consume or generate electricity. One chemical loses an electron (oxidation), whilst the other gains an electron (reduction). This is why they are called redox flow batteries, short for reduction-oxidation.
The killer feature of flow batteries is that to make your battery last longer, you can just increase the size of your tanks & chemicals - you don’t have to increase the size of your reactor. To get technical, you can scale energy and power separately.
This makes flow batteries attractive for long duration energy storage, because scaling the capacity of your battery doesn’t mean scaling the whole cost. Unfortunately, flow batteries are quite expensive to start with.
The leading type of flow battery uses an element called vanadium to store energy in the tanks.
Unfortunately, it’s not the cheapest element, and its production is highly concentrated. In 2022, China produced 70% of the world’s vanadium, and Russia 17%.
The price of vanadium is volatile, and usually quite high. Therefore, the costs of future vanadium flow batteries are estimated around $300 - 500 / kWh. It’s hard to imagine them competing with lithium packs at ~$150 / kWh, (or iron-air at $20 / kWh)
There are two things keeping people optimistic about flow batteries:
- A lithium battery for grid storage will probably last less than 10 years, but a vanadium flow battery battery can run for 20-30 years, and sometimes longer.
- Vanadium is not the only type of flow battery, and other electrolytes (chemicals in the tanks) are under development. There are countless combinations to explore.
Because of their simplicity, flow batteries are relatively easy to build as large scale experiments. There are iron flow batteries in Oregon, zinc-bromine batteries in California, and many more experiments worldwide. But with a cheap supply of vanadium, China leads the world with the largest installed flow batteries.
Against the backdrop of falling prices for lithium, sodium, and iron-air batteries, the future of flow batteries is less than certain. But in the wild west of batteries, nothing is off the table.
The end
Wow, that was a lot of battery.
Huge thanks to Nicholas Yiu and Lisa Wang for reviewing this monster piece and giving their invaluable thoughts 🙏
If you enjoyed reading this, please reach out! It helps me to know whether to write more like this.
Who's Ben James?
I spend my time deconstructing climate tech, and sometimes write down my thoughts on this blog. I’ve previously worked at World Fund, Octopus Energy, and the IPCC.
Sometimes I run training sessions for investors who want to study climate technologies in depth. Email ben at benjames.io.
Other writing
If you enjoyed reading this, you might like some of my other posts: